Thermoelectric Materials: A balancing challenge for recycling heat
By Jennifer Garland, Applied Physics & Math, 2021
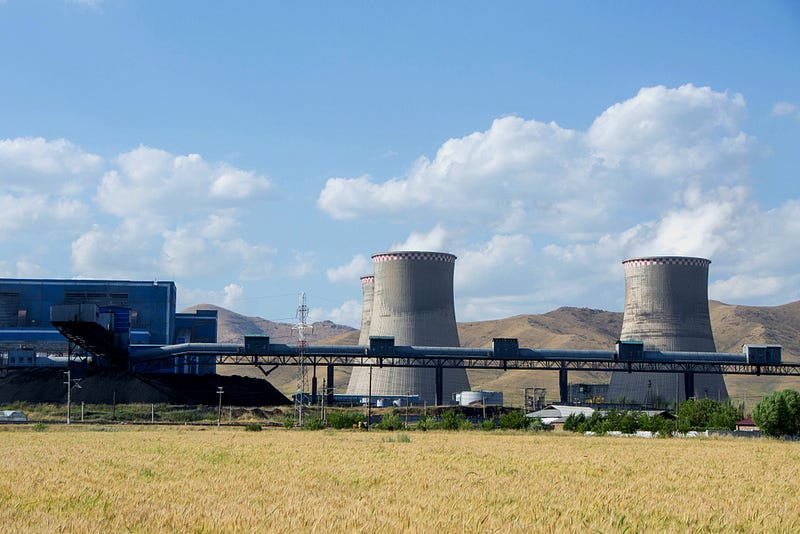
As the planet warms due to excessive burning of fossil fuels, and as these sources deplete, much focus has been on researching alternative energy sources. While developing these more sustainable technologies is vital, an often publicly overlooked source of energy is the waste heat from processes already in place, such as from power plants, appliances, and cars.
Unfortunately, thermodynamics limits efficiency to less than 50 percent in these systems, and all of the waste heat is simply given off into its surroundings. Figuring out a way to capture and recycle this extra energy would both reduce the problem of releasing heat into the environment and replace further electricity that would be produced from fossil fuels.
Figuring out a way to capture and recycle this extra energy would both reduce the problem of releasing heat into the environment and replace further electricity that would be produced from fossil fuels.
One way to utilize waste heat is through the Seebeck effect, which is a phenomenon where heat is converted into electricity. In some n- and p-type semiconductors called thermoelectric materials, the application of a temperature gradient causes current to flow. When one side of a thermoelectric material is cold and the other is hot, electrons move from the hot to the cold side, and electric potential builds up. However, finding suitable materials has proven a challenge over the last few decades.
Three main properties characterize a good thermoelectric material: high electrical conductivity, low thermal conductivity, and a high Seebeck coefficient (S). The Seebeck coefficient of a material is the amount of voltage resulting from the temperature difference across the material (S = –ΔV/ΔT), so it is desirable to have a high coefficient, indicating extracting the most electricity from the least amount of heat. High electrical conductivity allows for easier current flow, and low thermal conductivity retains the temperature gradient. However, these two transport properties usually come hand-in-hand, making it difficult to find optimal candidates.
The current leading thermoelectric material, bismuth telluride (Bi2Te3), is already used in some small-scale generators and cooling devices. Bismuth telluride has been utilized in bulk, but lower dimensional materials such as thin films and nanowires allow for further reduction of thermal conductivity, and therefore higher efficiencies. Alternately, modifying existing bismuth telluride systems by doping with semimetals has also been shown to improve transport properties.
The current leading thermoelectric material, bismuth telluride (Bi2Te3), is already used in some small-scale generators and cooling devices.
While bismuth telluride performs well at room temperature, the energy conversion is still limited to only 5 to 7 percent, and the elements are relatively rare and toxic, so more research is going into discovering and evaluating better materials. In September 2019, a study led by Wenke He of Beihang University was published in Science, presenting a new tin sulfide (SnS) based thermoelectric crystal that, if utilized, would reduce costs with earth-abundant elements.
Ultimately, the current efficiencies of the best-performing thermoelectric materials and generators are still too low to compete with existing power generation techniques. Teams like He’s are hard at work creating, altering, and testing new ones, in hopes that we can find a way to make inevitable heat loss do some work for us.
DOI: 10.1155/2018/1210562
DOI: 10.1126/science.aax5123