More Iron in a Biofilm’s Diet
By Hugh Shirley, Biochemistry, 2019
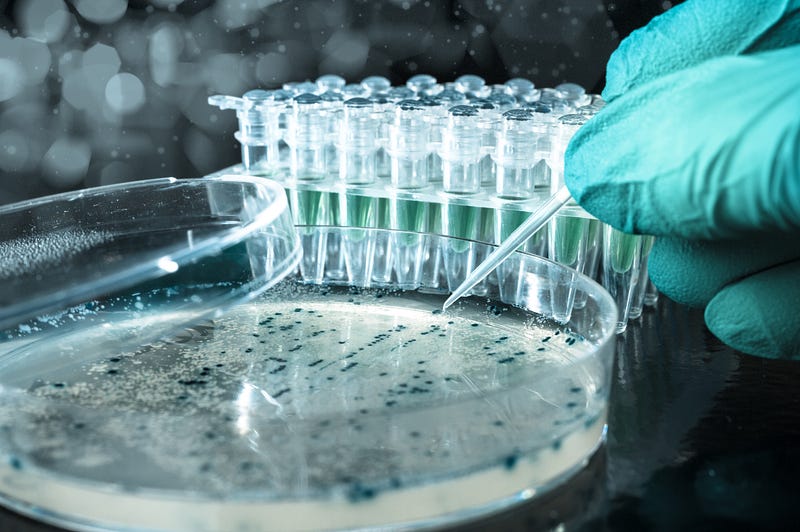
Biofilms are the name of the game in the Chai Lab at Northeastern University, and iron is shaking up the rules. Dr. Yunrong Chai and his team recently published an article in Nature Communications on their novel discoveries surrounding biofilm formation. Biofilms are communities of bacteria. They are tougher to kill with antibiotics than free bacterial colonies because of the protective coating that makes up the spaces between cells. The way that a biofilm forms and operates is still a question — one that Chai hopes to answer.
The team noticed that their model bacteria, Bacillus subtilis, requires hundreds of times more iron when growing as part of a biofilm than it needs to grow outside of a biofilm. This observation sparked the questions that started Chai and his team on their two-and-a-half-year-long project. Where is all that iron going, and what is it for? “Iron is very important, there’s lots of biological processes that need iron,” Chai said. “Bacteria will need iron to grow, and they will also need iron to build these biofilms.” Without all that extra iron, a robust biofilm will never form. Through a collaboration with the Larese-Casanova Research Group at Northeastern University, the team found that 99 percent of the iron needed for biofilm formation wasn’t used by the cells to grow and replicate.
Bacillus subtilis requires hundreds of times more iron when growing as part of a biofilm than it needs to grow outside of a biofilm. Where is all that iron going, and what is it for?
High iron concentrations induce transcription of genes that code for the precursor of a siderophore protein, called bacillobactin. Siderophores are a group of proteins that bacteria use to scavenge iron from their environments. The precursor, 2,3-Dihydroxybenzoate (DHB), solves a key problem with iron, its insolubility. DHB binds to iron and helps it dissolve in the aqueous environment of a biofilm. The theory is that iron then acts as an extracellular electron acceptor. The implications of that discovery are powerful, as it means that bacteria might be communicating in ways that we hadn’t previously realized.
Communication is key for biofilm formation; that’s because biofilms are complex, genetically identical bacteria that can differentiate into specialized, phenotypically diverse forms that carry out unique functions. If that sounds familiar, its because a biofilm is eerily similar to a multicellular organism. It was thought that chemical messengers were responsible for cell to cell communication. “Chemicals are the language of bacteria,” Chai said, but DHB changes things. Iron could act as part of a bacterial circuit that allows cells to communicate through electrical as well as chemical signals. That discovery isn’t the only conclusion to the biofilm story, however. A second use for extracellular iron could change what we’ve been taught about cellular respiration.
The electron transport chain (ETC) is the essential final step for aerobic respiration. Electrons are passed down a chain of membrane-bound proteins until they are deposited on an oxygen molecule, forming water. Iron can also act as the final electron acceptor instead of oxygen for energy production. It might not be as efficient as oxygen, but in the anaerobic depths of a biofilm, iron can be a valuable alternative. “The textbook always tells you that this always happens on the membrane,” Chai said. “They will never tell you that these electrons could leak and get into an extracellular space.” That is still electron transfer, according to Chai, even if it isn’t happening in a conventional way.
“The textbook always tells you that this always happens on the membrane. They will never tell you that these electrons could leak and get into an extracellular space.”
Extracellular electrical signaling and electron transfer for respiration aren’t two competing theories. According to Chai, these two processes are likely happening simultaneously. The Chai Lab found that disrupting these processes by preventing cells from producing DHB disrupted the whole biofilm.
Biofilms are found everywhere, from the bottom of the ocean to the driest deserts and even inside of our bodies. It’s theorized that biofilms in human and other eukaryotic microbiota might communicate directly with their hosts, potentially through Chai’s electronic mechanism. The Chai Lab’s paper represents a huge step towards a deeper understanding of how and why biofilms form, how they interact with their environments, and how they interact with us.
Nature Communications (2019). DOI: 10.1038/s41467–019–11681–0