Weird Flex: Field-Responsive Mechanical Metamaterials
By Jennifer Garland, Applied Physics & Math, 2021
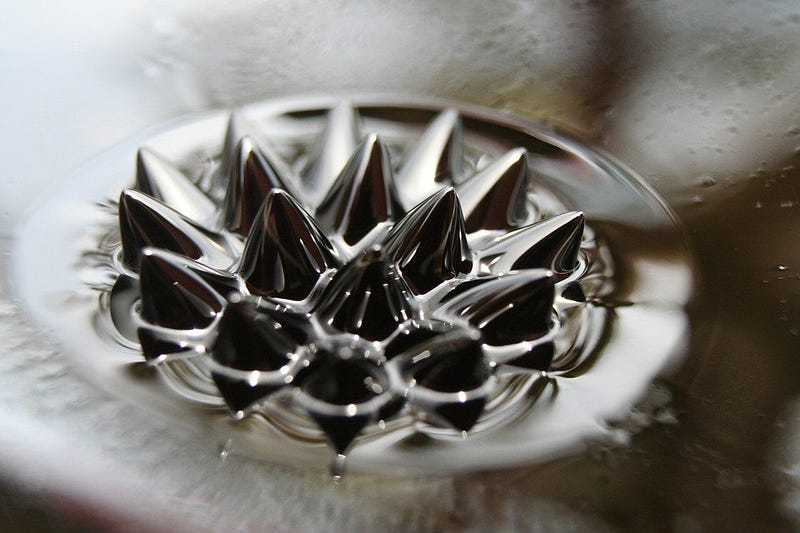
This article was originally published as part of Issue 39: Synthetic.
Many of today’s synthetic materials were inspired by nature. Jeffrey Grossman, a materials science and engineering professor at the Massachusetts Institute of Technology (MIT), has said that “nature is a polymer engineer gone wild.” However, even with all of the blueprints provided by nature, there is still demand for more efficient performance and increasingly technical applications. The field of metamaterials arose because scientists wanted to create materials with properties outside of nature.
Mechanical metamaterials, just one of many types, are structurally tuned to respond to forces in unconventional ways. This may mean that a material widens in one direction while being stretched in the perpendicular, the opposite of what a rubber band does, or that a solid responds to stress like a fluid: incompressible but not stiff. Recently, a collaboration led by engineer Julie A. Jackson at Lawrence Livermore National Laboratory (LLNL) introduced a new class of materials called field-responsive mechanical metamaterials (FRMMs) whose mechanical properties can be controlled by applying a magnetic field.
Mechanical metamaterials, just one of many types, are structurally tuned to respond to forces in unconventional ways.
The team first 3D-printed hollow polymer tubes that serve as the structural components of lattice shapes called cuboctahedrons. Then, the hollow lattices were injected with magnetorheological fluid, which is made up of magnetic microparticles suspended in liquid. This fluid acts like a normal liquid until a magnetic field is applied and the particles link up and align with the field, resulting in a large increase in viscosity. The aligned fluid looks similar to the spiky array of magnetized ferrofluid common in science museums, but the particles in ferrofluid are on the nanometer scale, much smaller than the microparticles. A photo of a cuboctahedron half-filled with magnetorheological fluid was featured on the cover of the December 2018 issue of Science Advances.
To demonstrate the effects of the increase in stiffness, the cuboctahedrons were placed under a load. Under a large applied magnetic field, the lattices supported the load, but as the field decreased, the structures began to lose stiffness and collapse. This behavior is significant because the structure of the material remained the same, but its mechanical ability was altered by the field.
This behavior is significant because the structure of the material remained the same, but its mechanical ability was altered by the field.
The development of FRMMs has been largely enabled by manufacturing innovations like 3D printing, especially a method called large area projection microstereolithography (LAPμSL) that was invented at LLNL. The researchers there will continue to optimize construction, ideally figuring out a way to integrate the magnetorheological fluid with the polymer lattice instead of having to manually inject it.
The level of control possible over these field-responsive mechanical metamaterials sets them apart from other mechanical metamaterials. The stiffness response in the LNLL experiments was very quick, less than a second, and that behavior is reversible without damage. Instead of just creating a material with useful set properties, FRMMs add a new dimension of customization controlled by a magnetic field. Because these materials may be applied to protective gear like helmets, having the ability to change according to environmental stimuli is critical.
This new class of metamaterials opens the door to dynamic components in a variety of areas. In addition to adaptive helmets, smart wearables that reduce vibrations, perhaps armor, could be on the way. Any application with movement control could benefit, such as car seats stiffening during a crash. Shoe soles have already incorporated mechanical metamaterials to improve impact absorption, and similar ideas can be applied to make buildings more earthquake-resistant or jet engines more thermally stable. With more testing, materials that stiffen up when needed most may soon be solidified.
Science Advances (2018). DOI: 10.1126/sciadv.aau6419